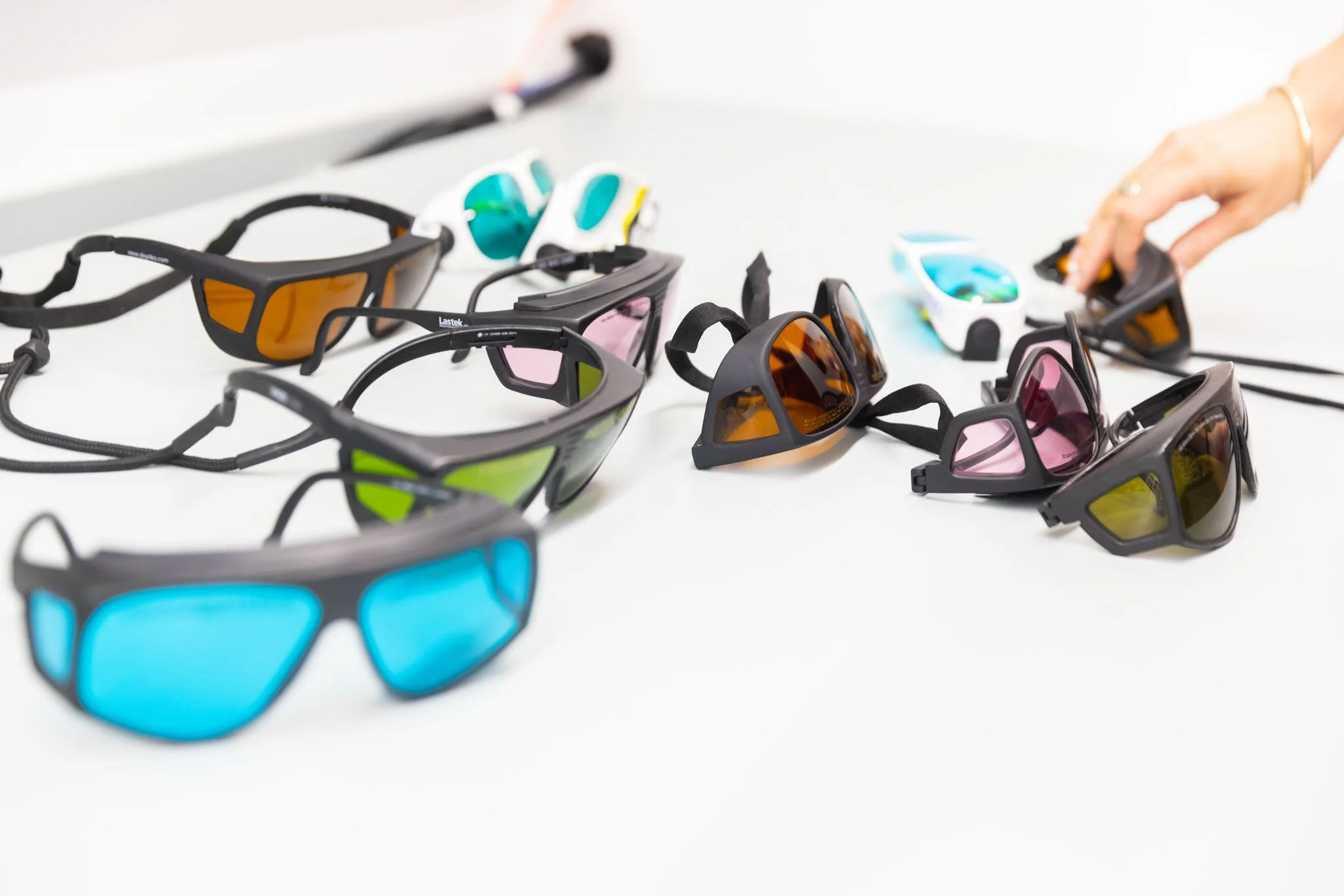
Join us!
Our lab offers diverse projects for students with physics or engineering backgrounds. Available projects are mostly of experimental nature, and typically aim to further our understanding of fundament quantum physics and superfluids. They also can include diverse technical aims, ranging from building optical setups, designing and realising electric circuits, optimising processes using machine learning to running experiments in one of our BEC apparatus.
Our lab is always looking for new members! A list of available research projects and positions can be found below. This is only a potential list of projects, please contact us to discuss additional possibilities.
Available PhD Projects
-
Our Q32 project seeks to improve Rydberg atom sensors for use near quasi-DC frequencies, while simultaneously boosting sensitivity through optical cavity enhancement. This PhD project will support this development and will include vapour cell fabrication and characterisation, along with engineering of the devices towards clinical applications.
-
Two-dimensional systems of vortices have emerged as a highly-controllable system for understanding the dynamics of long-range interacting particles. In particular, these systems appear poised to answer long-standing questions about the dynamics and phase transition characteristics in these systems. This project will focus on the precise control of vortices in our 2D superfluid systems, thoroughly investigating excitation spectra, defect persistence and spectroscopy, and measurement of phase transition characteristics.
-
Recent work in the UQ BEC lab has benchmarked new capabilities for the creation and manipulation of quantised vortices in quasi-2D superfluids. For example, the lab recently observed high-energy vortex clusters, associated with absolute negative temperature states of the vortex ensemble; for example see our publication in Science.
This project will expand the laboratory capabilities for producing arbitrary configurations of vortices and examining their dynamics. Deep analogies to the fractional quantum Hall effect, experimental understanding of the low-energy excitations of a vortex gas, and questions about vortex effective mass are all aims of this research direction.
Stipend: $32,192 per annum (2023 rate), indexed annually, with a $5k top-up available to exceptional candidates. -
Many of the turbulence and vortex experiments in the UQ BEC lab have focused on exquisite control and measurement of vortex dynamics in scalar BECs, which are BECs consisting of a single spin component. However, recent work in the UQ BEC lab has progressed to establishing a spinor condensate, consisting of all three spin states in the F=1 ground state of Rubidium 87. The capabilities of this experiment are being rapidly developed for the controlled creation of polar core vortices (PCVs) which are vortices in the spin texture of the spinor condensate but do not possess any overall fluid flow.
The aim of this PhD project will be to progress the study of PCVs dynamics beyond few vortex systems to more complex arrangements, building up spin turbulence from a bottom up approach. Additional aspects will be the application of spinor condensates to high-precision magnetic field sensing.
Stipend: $32,192 per annum (2023 rate), indexed annually, with a $5k top-up available to exceptional candidates. -
Atomtronics is an emerging field of applications of ultracold atom systems, where particle and superfluid properties can be used to realise analogs of conventional electronics or exploit additional degrees of freedom to realise novel circuit behaviour.
Some relatively recent work in the UQ BEC laboratory has demonstrated that the understanding superfluid circuit behaviour is improved through acoustic models. The aim of this PhD project will be to test a generalised acoustic model for more complex atomtronic circuits, especially one that incorporates the effects of dissipation and turbulence. Potential improvements to atomtronic devices, such as engineering acoustic resonances will be an additional aim of this project.
Stipend: $32,192 per annum (2023 rate), indexed annually, with a $5k top-up available to exceptional candidates.
Available Honours and Masters Projects
-
Bose-Einstein condensates (BECs) are an example of a superfluid system, described by a macroscopic wavefunction. The bulk of the superfluid is irrotational (zero circulation) and only poles in the wavefunction can support quantised amounts of circulation. These poles are referred to as quantised vortices and are of interest when studying quantum turbulence in BEC superfluids.
One difficult task when analysing these vortices is defining clustering in an objective manner. Cluster sorting can be considered a subset of the tasks appropriate for machine learning algorithms to solve. In addition, the experimental results available to us are images.
Therefore, image processing is also required which is another task suitable for machine learners. The aim of the project is to develop a complete machine learning algorithm to detect and graphically map quantised vortex clusters from single-shot images taken in the experiment. To accompany this, the algorithm will need to be error-tested using simulations of the system. Some research into the most appropriate algorithm will also be required.
-
The UQ Bose-Einstein condensation lab has highly developed methods for producing thin films of atomic superfluid and trapping and manipulating them using optical tweezers. The aim of this project is to investigate quantum vortices in the superfluid, which are like tiny whirlpools broadly similar to classical systems but possessing quantised angular momentum.
Building on recent successes in experimentally producing and measuring these vortices at UQ (https://arxiv.org/abs/2010.10049), this project aims to experimentally investigate the results of other UQ research that theoretically looked at the dynamics of pinning vortices in the BEC (https://arxiv.org/abs/2102.04712). Quantised vortices can be pinned (have their position fixed in place) by producing a density dip in the superfluid. This theory paper investigated the strength and dynamics of pinning, depending on the depth of the density dip.
This project will aim to investigate a proof of principle experiment, where the depth of a pinning potential will be modified and the relative velocity of the barrier required for depinning determined. The project will involve developing a control sequence for the optical trap for the BEC, based on existing software. The aim will then be to experimentally pin vortices and then determine the barrier velocity required for unpinning.
-
Solids, liquid, gas, plasmas, are known to most but at really low temperature a new exotic state of matter emerges: the Bose-Einstein condensate where all the atoms occupy the same state and behave like a macroscopic quantum wave. It has weird properties chief among them being irrotationality and frictionless like superfluids with phase coherent like a laser but is made up of atoms instead of photons. This allows for the investigation of quantum effects at a macroscopic level!
The Bose-Einstein Condensate (BEC) Laboratory at UQ uses lasers and magnetic traps to contain and cool atoms down to the Bose-Einstein condensate state of matter. We explore problems of fundamental physics such as investigating turbulence in superfluids and quasiparticle systems in negative absolute temperature regimes and their consequence for underlying host physical systems. We produce emulations of other quantum and classical systems, such as pulsar glitches and the big red spot on Jupiter, and perform precision sensing, focusing on the measurement of rotation and magnetometry.
-
Neutron stars are astrophysical laboratories, realising extreme states of matter far beyond what might be created on Earth. Among the mysteries of neutron star behaviour are rotational glitches in radio pulsars – a sudden spin-up of the neutron star. Since direct observation of the microscopic processes leading to glitches is essentially impossible, laboratory analogues could favourably be used to elucidate the physics at work. This project will realise a laboratory neutron star by using a superfluid Bose-Einstein condensate. This system will be used to investigate depinning avalanches of quantised vortices – a key candidate mechanism in neutron star glitches.
-
Dissipation of energy from the superfluid by the uncondensed thermal cloud plays a major role in the dissipation of superfluid excitations. Recently, results with degenerate fermi gases have hinted at anomalous behaviour in the dissipation of superfluid vortices. The aim of this project is to use our precision control over the 2D superfluid to create and precisely determine the mutual friction parameters driving dissipation in a bose superfluid. We aim to compare these results with those of a degenerate fermi gas system.
Undergraduate Projects (including Capstone)
-
Imaging of Bose-Einstein condensates requires high-sensitivity detectors and images are often dominated by un-scattered light, complicating the detection of fine microscopic features such as quantised vortices and phonons (sound waves). Thanks to the Fourier transforming properties of optical diffraction there is a better way – throw away most of the unscattered light and keep the signal you want. Surprisingly, this spatial- frequency Fourier filter can be implemented with some simple optics, consisting of a couple of lenses and a small blocking mask. In effect, the concept is to produce a Photoshop-style edge detection filter, but rendered real-time and analogue by physical optics and a bench top optical system. This technique is known as darkground imaging.
The aim of this project is to explore and characterise the implementation of darkground imaging via a benchtop optical system, and determine its efficacy and capabilities. Opportunity to implement the system on the UQ Scalar BEC apparatus may arise towards the end of the project.
-
Atoms must be trapped to create a Bose-Einstein Condensate (BEC). This can be done either optically or magnetically. Optical trapping is insensitive to the magnetic sublevel of an atom, whereas in a magnetic trap, the degeneracy of these different states is lifted. Using optical traps one can create BECs in any internal state, allowing the spin of the atoms to be essentially a free parameter. Spinor condensates, which are multi-component condensates can be created in optical traps, where all magnetic sublevels of an internal state are populated. Adding spin freedom to our emulator increases parameter space which can be explored. The populations of these states can be altered passively through spin-exchange collisions or actively through time-varying magnetic fields (typically radio frequency or microwaves). By proper application of these fields, we can prepare the condensate in mixtures or superpositions of various internal states through spin flips.
This project will quantify and develop the methods, such as Rapid Adiabatic Passage, to couple and prepare desired internal state mixtures. This will then be applied to our condensates to study the dynamics and superfluid behaviour of spinor condensates in these systems.
-
Creating Bose-Einstein condensates requires lasers that are locked to particular atomic transitions. To do this, we typically scan the frequency of the laser and pass the light through a cell containing the a vapour of the atom of interest (in our case, rubidium). By measuring the light intensity on the other side of the cell, we can then detect the precise frequency at which the absorptions occur which correlate to these transitions.
An alternative approach to this method would be to instead, keep the laser locked to a particular frequency and apply an AC magnetic field to the absorption cell to scan the transition frequency through Zeeman shifting.
This project aims to setup the laser locking using these two methods and determine which one gives the most stable lock onto atomic transitions. The preferred setup will then be integrated into our Bose-Einstein condensate experiment.
-
Ultracold atoms are trapped in magnetic and optical traps. Trapping of ultracold atoms and condensing them into a Bose-Einstein condensate (BEC) allows us to study many quantum mechanical phenomena, such as the superfluid fountain effect and clustering of quantum vortices, leading to local negative absolute zero temperatures.
One method to optically trap ultracold atoms is in an optical lattice: Through interference of two optical beams, we create an accordion-shaped set of fringes in which we trap our atoms. While this method allows us to create very pure BECs, the downside of using optical interference is that it is very susceptible to phase fluctuations of the laser beams, mainly induced through vibrations. These vibrations shake the atoms inside the lattice heating them and reducing the lifetime of the BEC.
The aim of this project is to design and test a feedback system that is robust against any mechanical noise. The project includes a literature review of successful feedback schemes for optical lattices, programming a microcontroller to realise a feedback system, and setting up a proof-of-principle stabilised optical lattice in our lab.
-
Bose Einstein condensates (BECs) are used to study quantum effects at the macroscopic scale. This has allowed BEC systems to be developed into quantum simulators and extremely sensitive sensors for applications like the search for dark matter.
To do this, it is necessary to have a very high level of control over the parameter space of the atoms involved. In the UQ BEC lab, we achieve this control by projecting arbitrary light patterns onto the condensates and have managed to create optical potentials which trap the atoms with resolutions of about 1 μm (close to the diffraction limit of the light used). This does however mean that we are very susceptible to small changes in environmental conditions, leading to a lot of time being spent focussing optics to maintain this resolution.
The project aims to improve this process by implementing autofocusing of lenses that project these light potentials using computer interfaced lens stages. It will involve implementing the preexisting software to control these lenses, developing a way of determining when optimal focusing has been achieved and then feeding back to alter the lens position.
Postdoctoral Positions
None currently open — please check back soon!